- Composite Biomaterials for Tissue Engineering and Regenerative Medicine
- Directed Differentiation of Stem Cells
- Ultrasound-based Characterization of Soft Biomaterials (with Cheri Deng)
Composite Biomaterials for Tissue Engineering and Regenerative Medicine

We are developing composite biomaterials consisting of the proteins collagen I and fibrin, which are simultaneously polymerized to produce interpenetrating polymer networks. By embedding cells directly into such matrices at the time of polymerization, we produce rudimentary tissues. A main focus of this project is to develop mechanistic models of how such composites lead to increased mechanical properties, by combining detailed characterization of the microstructure of these matrices with macroscopic materials testing. A second arm of this project examines how cell function is altered in such 3D matrices. We examine changes in gene and protein expression in response to changes in composite structure, and link these changes to intracellular signaling events. Our goal is to determine which key signaling pathways are involved in controlling cell function in 3D matrices, and to design environments that can regulate these pathways.
The mechanical testing techniques we use include conventional compressing and tensile testing, as well as viscoelastic properties using dynamic mechanical analysis, creep recovery and stress relaxation tests. In addition, we have initiated collaborations on multiscale computational modeling of our materials, and are investigating the addition of other polymers in the matrix, as well as the application of defined mechanical strain. We have developed initial mechanistic models of how protein composite materials behave under stress and how they fail, as well as how the mechanical properties and protein composition affect cell function. These conceptual models provide a framework from which we can generate testable hypotheses.
Directed Differentiation of Stem Cells
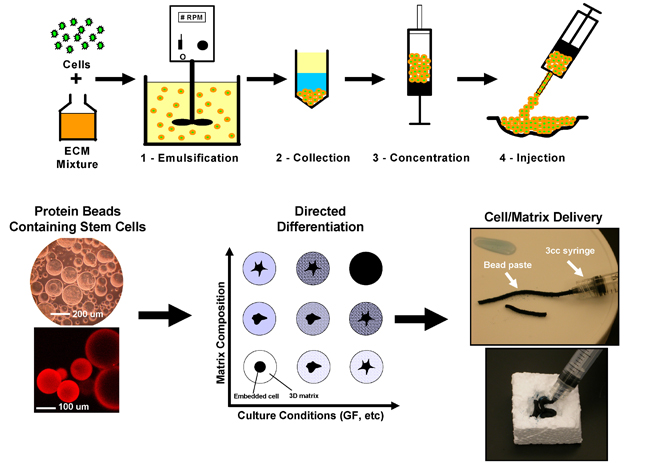
This project involves embedding stem cells (currently we use adult human mesenchymal stem cells, hMSC) directly in protein-based hydrogel beads of 50-200 micron diameter. This allows us to tailor the protein composition of the bead in order to promote differentiation of the embedded hMSC toward a desired lineage. The advantage of the bead format is that it requires only small amounts of protein, and the beads can be concentrated and handled as a slurry or paste, such that beads can be delivered directly to a site of injury. Our current focus is on osteogenic differentiation of hMSC for the purpose of bone repair. We have developed a variety of bead formulations using composites of collagen, vitronectin, fibronectin, gelatin, chitosan, and agarose. We also are extending this technology to the enhancement of stem cell engraftment in the heart after transplantation.
The technology of making defined 3D microenvironments from biologically-relevant proteins and polysaccharides has potentially broad application. In addition to the orthopedic and cardiovascular applications currently being pursued, this technique can be applied to other tissue types in which the cellular microenvironment is of particular importance, for example cartilage and neural tissue. This project also can be extended to other types of stem cells, in which the cellular niche is known to control cell fate. Because the bead format offers a way to deliver cells while still encased in the appropriate protein environment, we have begun development of a minimally invasive delivery system and we have initiated pilot studies on the use of protein-based beads for the suspension culture of anchorage-dependent mammalian cells.